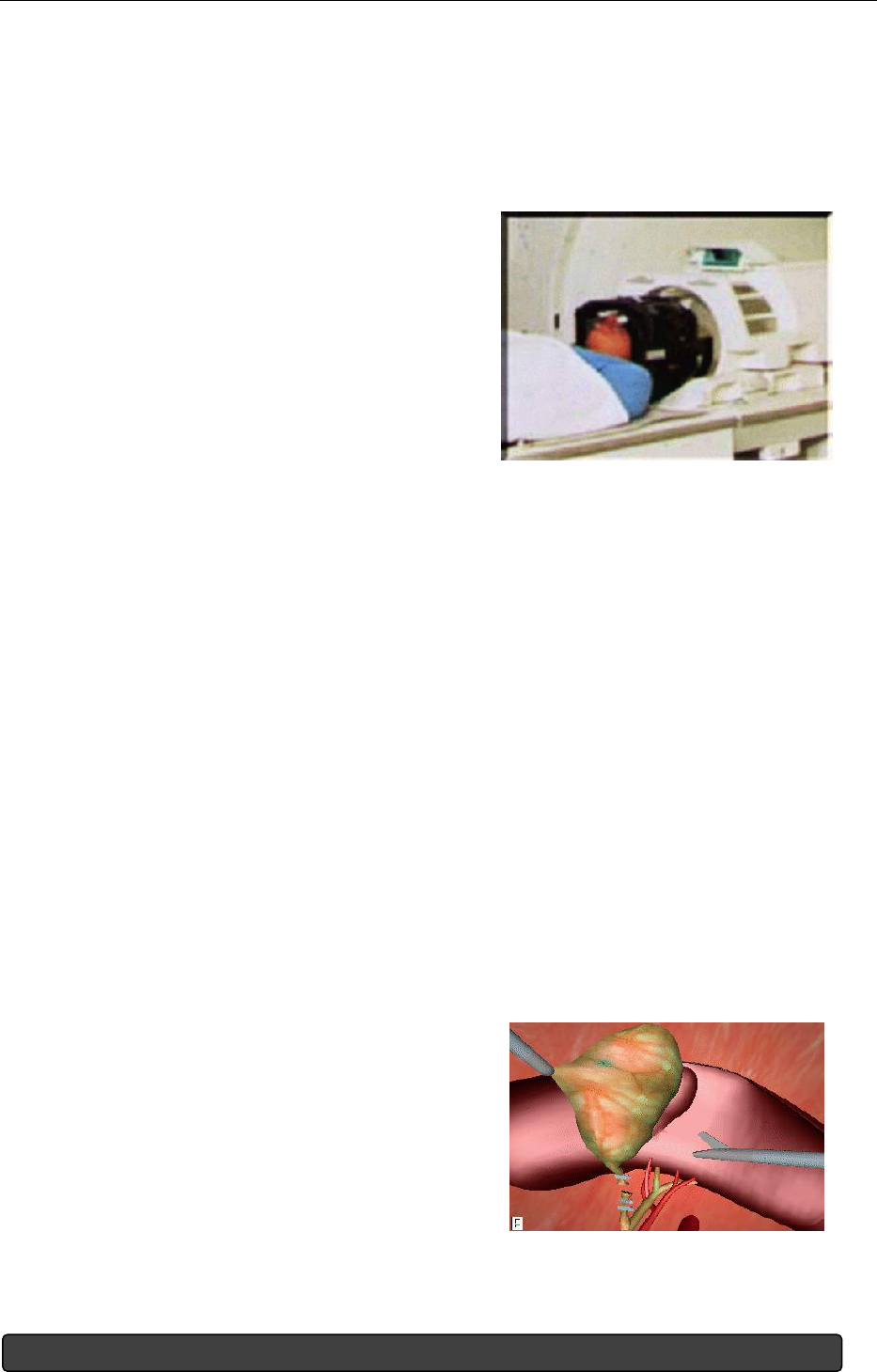
S.S Chopade et al. Int. Journal of Engineering Research and Applications (IJERA) www.ijera.com
Vol. 3, Issue 5, Sep-Oct 2013, pp.247-251
www.ijera.com 248 | P a g e
III. Applications in Medicine
Robots are filling an increasingly important
role of enhancing patient safety in the hurried pace of
clinics and hospitals where attention to details and
where reliability are essential. In recent years, robots
are moving closer to patient care, compared with their
previous role as providing services in the
infrastructure of medicine. Examples of past use are in
repetitive activities of cleaning floors and washing
equipment and carrying hot meals to patients‟ bedside.
What is new is finding them in clinical laboratories
identifying and measuring blood and other specimen
for testing, and in pharmacies counting pills and
delivering them to nurses on „med-surg-units‟ or
ICU‟s. Or bringing banked blood from the laboratory
to the ED, surgery or ICU for transfusions. Robots are
being used as very accurate „go-fors‟!
An early active robot, „Robodoc‟ was designed to mill
perfectly round lumens in the shafts of fractured
bones, to improve the bonding of metal replacements
such as for femur heads and knee joints. The future of
this system remains uncertain because of questions
about the ultimate beneficial outcomes.
The reasons behind the interest in the
adoption of medical robots are multitudinous. Robots
provide industry with something that is, to them, more
valuable than even the most dedicated and hard-
working employee - namely speed, accuracy,
repeatability, reliability and cost-efficiency. A robotic
aid, for example, one that holds a viewing instrument
for a surgeon, will not become fatigued, for however
long it is used. It will position the instrument
accurately with no tremor and it will be able to
perform just as well on the 100
th
occasion as it did
on the first.
IV. Robotic surgery
Robotic surgery is the process whereby a robot
actually carries out a surgical procedure under the
control of nothing other than its computer program.
Although a surgeon almost certainly will be involved
in the planning of the procedure to be performed and
will also observe the implementation of that plan, the
execution of the plan will not be accomplished by
them - but by the robot.
In order to look at the different issues involved in the
robotic fulfillment of an operation, the separate
sections of a typical robotic surgery (although robotic
surgery is far from typical) are explained below.
Surgical planning
Surgical planning consists of three main
parts. These are imaging the patient, creating a
satisfactory three-dimensional (3D) model of the
imaging data, and planning/rehearsing the operation.
The imaging of the patient may be accomplished via
various means. The main method is that of computer
tomography (CT). CT is the process whereby a stack
Of cross-sectional views of the patient are taken using
magnetic-resonance-imaging or x-ray methods. This
kind of imaging is necessary for all types of operative
procedure and, as such, does not differ from traditional
surgical techniques.
Fig 1: A patient having a brain scan
This two-dimensional (2D) data must then be
converted into a 3D model of the patient (or, more
usually, of the area of interest). The reasons for this
transformation are twofold. Firstly, the 2D data, by its
very nature, is lacking in information. The patient is,
obviously, a 3D object and as such, occupies a spatial
volume. Secondly, it is more accurate and intuitive for
a surgeon, when planning a procedure, to view the
data in the form that it actually exists. It should be
noted, however, that the speed of said hardware is
increasing all the time and the price will decrease too,
as the technology involved becomes more
commonplace. This means that the process will be
more cost-efficient and increasingly routine in the
future.
The third phase of the planning is the actual
development of the plan itself. This involves
determining the movements and forces of the robot in
a process called „path planning‟ - literally planning the
paths that the robot will follow.
Fig 2: A surgery simulation to aid planning