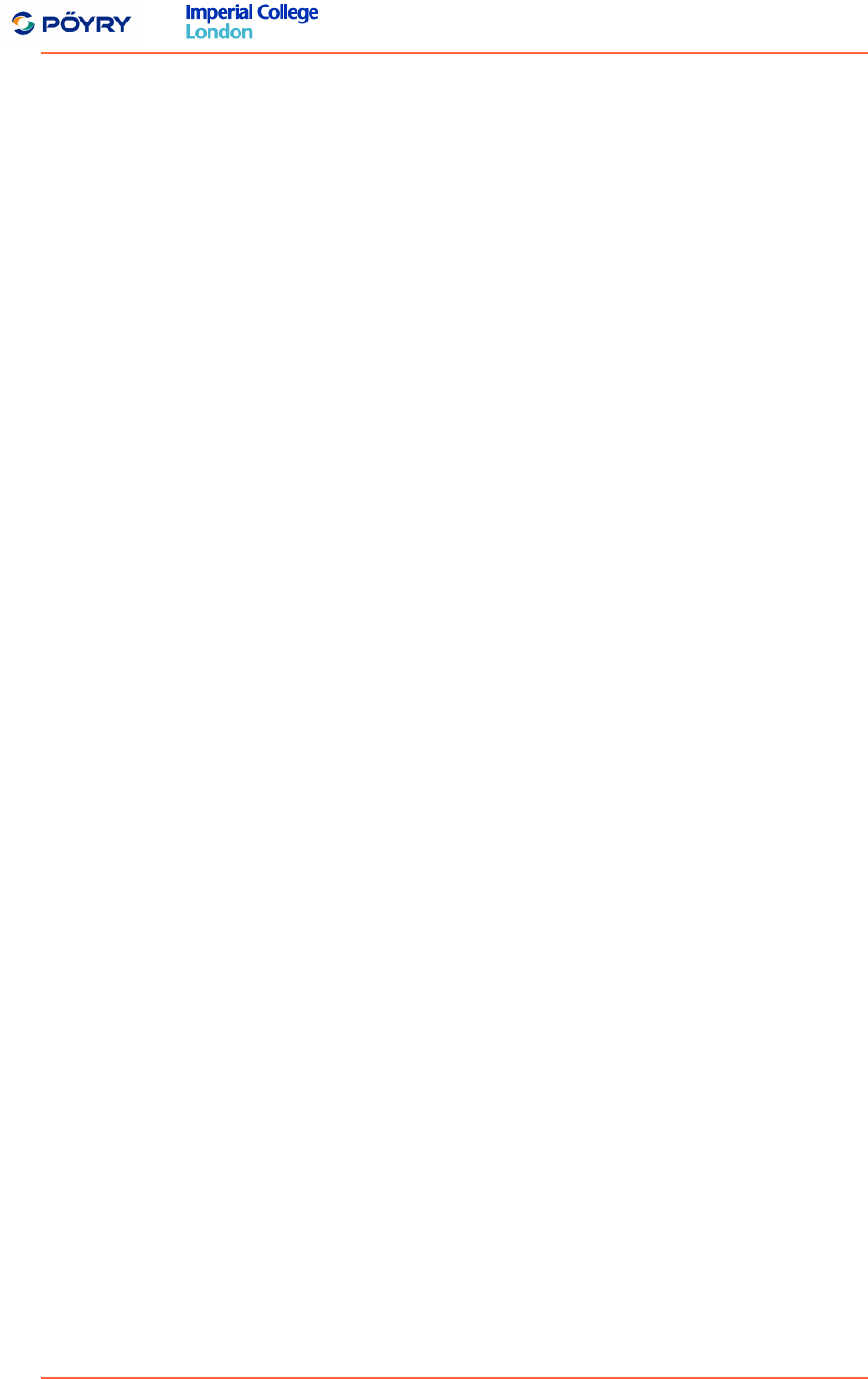
ROADMAP FOR FLEXIBILITY SERVICES TO 2030
May 2017
379_FlexibilityRoadmap_FinalReport_v200.docx
69
PÖYRY MANAGEMENT CONSULTING
IMPERIAL COLLEGE LONDON
The following assumptions regarding demand side flexibility are made in the system
modelling analysis:
electric vehicles: up to 80% of EV demand could be shifted away from a given hour to
other times of day;
heat pumps: heat storage enables that the 35% of HP demand can be shifted from a
given hour to other times of day;
smart appliances: demand attributed to white appliances (washing machines,
dishwashers, tumble dryers) participating in smart operation can be fully shifted away
from peak;
industrial and commercial (I&C) demand: 10% of the demand of I&C customers
participating in DSR schemes can be redistributed; and
daily consumption: the modelling assumes that the demand side response will not
change the daily energy consumption.
In addition to improving energy management and potentially reducing generation and
network capacity requirements due to reduced peak demand, these flexible demand
sources are also assumed to be capable of providing ancillary services – for example:
smart fridges can provide frequency regulation as it detects the frequency deviation and
reduces the load when the frequency is low as long as the temperature in the fridge is still
within the permissible limits. Electric vehicles can also temporarily interrupt their charging
if the system frequency is low. This simple control mechanism can provide substantial
frequency response services to the system at low costs. As the frequency of the events
that trigger the utilisation of this service is relatively low, and the duration of having low
frequency is relatively short because the system operator will restore the system
frequency back to nominal levels within minutes, this will not substantially change the daily
operation of the flexible load devices.
Investigation of the Impact of Electrifying Transport and Heat Sectors on the UK Distribution
Networks, C.K. Gan, M. Aunedi, V. Stanojevic, G. Strbac and D. Openshaw, 21
st
International Conference on Electricity Distribution (CIRED), Frankfurt, Germany, 6-9 June
2011
Smart control for minimizing distribution network reinforcement cost due to electrification”, D.
Pudjianto, P. Djapic, M. Aunedi, C. K. Gan, G. Strbac, S. Huang, D. Infield, Energy Policy,
Vol. 52, pp. 76-84, January 2013
Value of Smart Appliances in System Balancing, Part I of Deliverable 4.4 of Smart-A project
(No. EIE/06/185//SI2.447477), Imperial College London, September 2009
Economic and Environmental Benefits of Dynamic Demand in Providing Frequency
Regulation, M. Aunedi, P. A. Kountouriotis, J. E. Ortega Calderon, D. Angeli, G. Strbac,
IEEE Transactions on Smart Grid, vol. 4, pp. 2036-2048, December 2013
Distributed generation and demand response services for the smart distribution network, M.
Woolf, T. Ustinova, E. Ortega, H. O’Brien, P. Djapic, G. Strbac, Report A7 for the “Low
Carbon London” LCNF project, Imperial College London, 2014.
Understanding the Balancing Challenge, analysis commissioned by DECC, Imperial College
and NERA Consulting, 2012
https://www.gov.uk/government/uploads/system/uploads/attachment_data/file/48553/5767-understanding-the-balancing-challenge.pdf
An overview of the rationale and evidence behind these assumptions is provided in ‘Carbon
impact of smart distribution networks’, M. Aunedi, F. Teng, G. Strbac, Report D6 for the “Low
Carbon London” LCNF project, December 2014